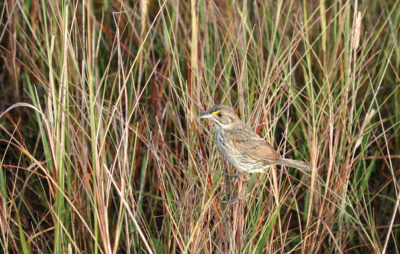
Figure 1: A Cape Sable seaside sparrow perched on sawgrass in the Everglades National Park. Photo courtesy of National Park Service.
The Cape Sable seaside sparrow, Ammodramus maritimus mirabilis (Figure 1), one of nine surviving subspecies of seaside sparrows (the Dusky seaside sparrow was declared extinct in 1990), is endemic to southern Florida and is listed as endangered under the current Endangered Species Act1. While the subspecies of the seaside sparrow are distributed generally along the east coast of the U.S. (Figure 2), from Massachusetts to southern Florida, and along the Gulf coast, from southeast Texas to the west coast of Florida, the Cape Sable seaside sparrow is found primarily in the Everglades National Park (ENP).
Editor’s note: A PDF of this article as it appeared in the magazine is available HERE.
A key element for the Cape Sable seaside sparrow’s survival is the short hydroperiod pattern that maintains the wet prairie plant community. The preferred nesting habitat of this ground-nesting species appears to be a mixed marl prairie community that often includes muhly grass. The birds build cup-shaped nests about 10 – 12 centimeters (cm) above the ground and, hence, the nests are subject to flooding when water depths reach above 10 cm. Pimm et. al.2 suggest that nesting will not be initiated if water levels are at a depth greater than 10 cm during the spring breeding season. The normal hydroperiod, consisting of regular but brief rainfall events, usually maintains the surface water levels at or below the ground-level.
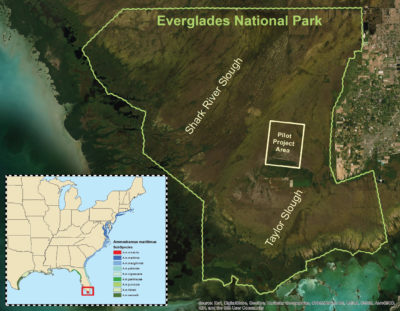
Figure 2: Everglades National Park and pilot project area; inset shows distribution of extant sub-species of the seaside sparrow.
The Cape Sable seaside sparrow’s short hydroperiod wet-prairie habitat is contained entirely within the Central and South Florida Project’s 4.6-million hectare footprint, which includes ENP (Figure 2). The two major Cape Sable seaside sparrow sub-populations occur within the park; one west of Taylor Slough and the other west of Shark River Slough in the Big Cypress National Preserve (Figure 2). The Everglades Multi-Species Recovery Plan for South Florida (2019 revision)3 identified several areas for study to enhance the breeding habitat for the birds. Special attention was given to (1) altering current water management practices, (2) studying the existing breeding habitats, and (3) potentially restoring additional habitats. However, without accurate topographic maps of ENP, managing the hydroperiod and restoring additional habitat for breeding would not be possible. In the wet prairie, the ability to measure shallow water depths and small changes in topography is critical to the ENP’s effort in restoring nesting habitat for the Cape Sable seaside sparrow.
Enter topobathymetric lidar sensors
Conventional airborne lidar technology was developed using lasers in the near infrared range—1064 nanometers (nm)—of the spectrum to measure topography. As a result, the laser pulses are of long duration and are nearly totally absorbed by water, or, at best, only the nadir pulses over water are returned to the sensor. By frequency-doubling the laser to 532 nm (within the visual green range of the spectrum), adjusting the emission angle and decreasing the pulse duration from the emitter, laser pulse penetration through water, and hence bathymetry, was achieved. These “topobathymetric” lidar sensors are ideal for mapping through the water and emergent vegetation in ENP. Most critically, the green lasers in combination with infrared lasers also measure the water surface and can identify and measure areas, when wet, that do not exceed the critical 10 cm water depth for Cape Sable seaside sparrow breeding.
In 2016, the U.S. Geological Survey (USGS) and the National Park Service (NPS) contracted with Dewberry to perform a topobathymetric survey of the 1211 square miles of the ENP (Eastern Everglades) in south Florida (Figure 2). The project specifications followed the USGS Lidar Base Specifications 1.2 for Quality Level 1 (Table 1):
Dewberry is unique among lidar-data providers, in that the firm does not own or operate any sensors: with multiple different topobathymetric lidar sensors available through sub-contractors, it could choose the best sensor for the ENP project. After narrowing down the options to two sensors, Dewberry suggested a novel approach to NPS—perform a pilot project using both sensors flown over the same area to make the decision. USGS and NPS agreed to the plan, a 33-square-mile pilot area was selected (Figures 2 and 3), and two independent lidar acquisition missions were planned (Table 2):
The pilot project
In collaboration with ENP, Dewberry identified a pilot project area to include as many different habitat types as possible for testing the lidar sensors’ ability to model the terrain. After consideration, four habitats, (1) upland forest, (2) tree island/marsh, (3) retention pond (open water), and (4) freshwater slough/prairie, were chosen as representative habitats, including critical vegetated wet prairie, marsh, and tree island habitats for nesting seaside sparrows. The 33-square-mile area of interest (AOI) (Figure 3) was selected and the pilot project acquisitions were performed between May and June of 2016. The National Oceanographic and Atmospheric Administration (NOAA) conducted the VQ-880-G mission and Leading Edge Geomatics conducted the Titan mission.
Pilot project—results
To analyze and compare the lidar data from the two sensors, Dewberry used Esri ArcGIS Desktop with the 3D Analyst extension. Classified lidar (LAS) data were built into LAS Datasets and Esri Terrains were constructed for visualization and analysis. Bare-earth digital elevation models (DEMs) were extracted from the Terrains to visualize comparisons.
As the goal of the pilot project was to assess the sensors’ ability to discern breeding habitat for the Cape Sable seaside sparrow, the critical components included: (1) each sensor’s ability to model ground measurements at a sufficient density to be confident of the DEMs constructed to predict suitable habitat, and (2) the ability of the sensor to accurately measure the ground through dense vegetation, tree islands, and marshes to assess the 10 cm water depth.
With regard to pulse density, while both sensors were capable of greatly exceeding USGS specifications for QL1 lidar data, the VQ-880-G sensor recorded 60% more green (532 nm) returns than the Titan over the pilot area (Table 3). Notably, the Titan, with two near-infrared (NIR) channels operating at a higher pulse frequency than the VQ-880-G, recorded almost five times the number of NIR pulses as did the VQ-880-G (Table 3):
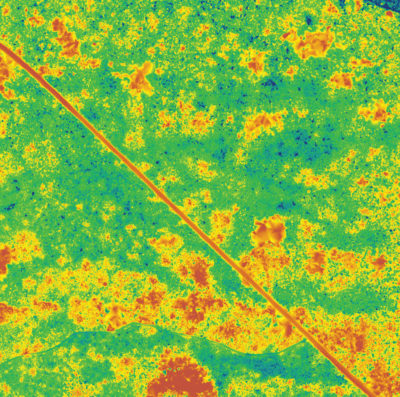
Figure 4: DEMs (bare earth) constructed from green returns from VQ-880-G (above) and Titan (below) in heavily vegetated areas in the ENP (lower elevations in blue; higher elevations in red). The distinctive linear feature from northwest to southeast is an elevated hiking trail.
To evaluate the sensors’ performance in critical breeding habitats, DEMs were generated from the green and NIR channels in heavily vegetated and tree island/marsh habitats. The DEMs constructed in the heavily vegetated areas were very similar (Figure 4) with some minor differences that were attributed to acquisition date. In the tree island/marsh habitat, profiles from the VQ-880-G data revealed returns through the vegetated canopy on to bare earth under vegetation, particularly in the wet prairie/marshy areas. The Titan data showed fewer returns through the vegetated canopy and less dense data in bare earth in the wet prairie/marshes. The VQ-880-G data appear to penetrate through the wet prairie/marsh to produce lower ground elevation returns as compared to the Titan, in some areas up to 60 cm lower (Figure 5).
Pilot project—conclusions and recommendations
After using conventionally surveyed ground check points to assess the horizontal and vertical accuracy, and measuring the aggregate nominal pulse density, Dewberry found that both sensors greatly exceeded the USGS specifications for QL1 lidar data. However, the ground modelling capabilities of the two sensors exhibited subtle differences in tree island and wet prairie/marshes, both critical habitat for Cape Sable seaside sparrow breeding activities.
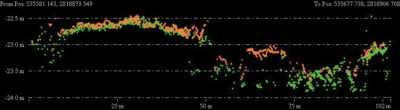
Figure 5: Profile view through a tree island and associated marsh showing VQ-880-G 532 nm data (green points) and Titan 532 nm data (orange points).
Based on the above analysis, Dewberry recommended:
- Using a topobathymetric lidar sensor, rather than a topographic-only lidar sensor, for the project,
- Using a sensor with a short-pulse, green (532 nm) laser and a NIR (1064 nm) laser to map the ENP
- Increasing operating altitudes to 600-750 meters above ground level (AGL) would meet the USGS QL1 density, pulse-spacing and vertical accuracy specifications. The higher operating altitude will enable a more cost-effective solution.
The rest of the story
Following the successful pilot project in 2016, Riegl short-pulse duration sensors were used to map 1211 square miles of the eastern ENP (Figure 2). This acquisition was accomplished between April and June 2017. The data were delivered to and accepted by USGS/NPS in October 2018. These data are currently available on the US National Map and being used by the ENP to identify critical habitat for Cape Sable seaside sparrows.
Dewberry is currently mapping the western ENP AOI (Figure 6). This additional 788-square-mile project, with potential Cape Sable seaside sparrow breeding habitat, was surveyed between March and June 2019, using the VQ-880-G sensor, and is anticipated to be available for distribution in fall 2020, both as part of the US National Map and on the NOAA Digital Coast.
Acknowledgements:
We thank the USGS and the NPS/ENP for their assistance with this project. Ryan Ligon (Dewberry) was instrumental in navigating to ground check points in the Everglades.
For more information, contact Keith Patterson (see below).
2 Pimm, S.L., K. Balent, T. Brooks, J. Curnutt, T. Fenn, N. Fraley, S. Killeffer, J. Lockwood, L. Manne, A. Mayer, M.P. Nott, G. Russell, and E. Stanton, 1996. Population ecology of the Cape Sable seaside sparrow, draft report. Unpublished report prepared for the U.S. National Park Service and the U.S. Fish and Wildlife Service, Vero Beach, Florida.
3 South Florida Multi-Species Recovery Plan, 2019. https://www.fws.gov/verobeach/ListedSpeciesMSRP.html.